The first rule of Clock Club is: you cannot stop talking about Clock Club.Last week I was privileged to spend four days attending the 43rd Annual Time & Frequency Metrology Seminar at the National Institute of Standards and Technology at their laboratories in Boulder Colorado. NIST is one of the premier scientific and technology standards organizations in the world (they were formerly the National Bureau of Standards), and continually produces bleeding edge breakthroughs, many of which end up becoming commercialized into mainstream technology that changes our lives for the better.
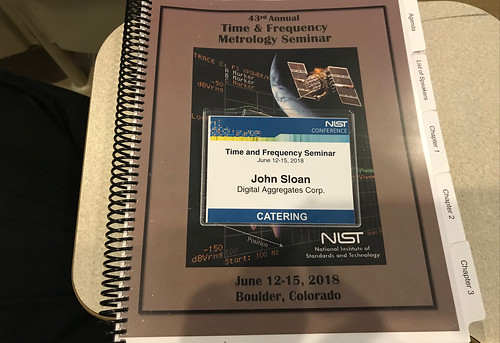
Here are some Fun Facts To Know And Tell on the material presented by NIST scientists as part of the Time and Frequency Seminar, and some photos of stuff I saw. Any bone headed remarks are strictly my fault, and are probably the result of my being just a humble software developer instead of a research physicist or an electrical engineer, or maybe just not taking good notes.
My electrical engineer and physicist friends would certainly have gotten even more out of this seminar than I did. Most of the people there were EEs or physicists, from other national labs, other time standards organizations, from commercial atomic clock manufacturers, etc. For example: one guy from the National Physics Laboratory (NPL), the U.K. equivalent to NIST; a woman who was a lieutenant in the Spanish navy (had served on a frigate) now working at Spain's Naval Observatory (which has the same role as the U.S. Naval Observatory, which provides the master time reference for the U.S. military, and is a reminder that our definition of time is central to navigation at sea and is still in part in the province of astronomers); a guy who worked for the Canadian Department of National Defense (equivalent to the civil service portion of the U.S. Department of Defense) in the organization that is responsible for instrument testing and quality, etc.
Oh, and some guys whose badges listed no organization, but wrote "DOD" on a sign up sheet, and told me they were from "Maryland". Past experience tells me they work for an intelligence agency. This is not an unusual encounter in my line of work; it happened all the time when I attended conferences while employed at the National Center for Atmospheric Research due to our common interests in high performance computing and mass storage systems. The pink I. M. Pei-designed NCAR Mesa Laboratory where I worked is barely visible in the photograph below in the distance above and to the right of the NIST Building 1 where the seminar was held.
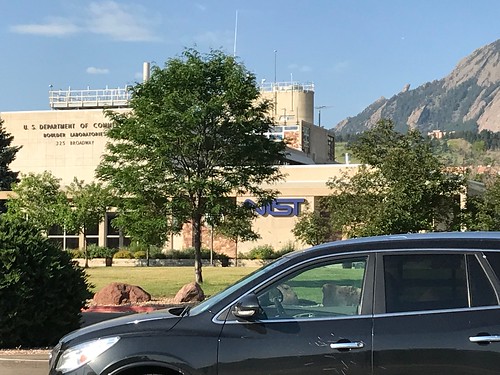
The phrase I learned that I will be most likely to drop into conversations in the future: "clock trip". I knew this was done, but I didn't know this is what it was called: you synchronize/syntonize/phase-lock a rack-mounted commercial atomic clock, running on a big battery pack, to an atomic clock at one laboratory, then you put it on a cart/truck/plane/ship/etc. and take it to another laboratory, maybe far away, where you compare it to another atomic clock. This is old school, but it still done from time to time today, since it yields the best results. Mostly, "time transfer", as this procedure is generically known, is also done over dark fiber, geosynchronous satellites, or, most typically today, GPS. Everyone now compares their atomic clocks to GPS Time (GPST), which is synced ultimately to International Atomic Time (TAI).
NIST generates UTC(NIST) time. About once a month or so, the International Bureau of Weights and Measures (BIPM) in Paris publishes an announcement of how far UTC(NIST), and every other "national" time standard, diverges from standard Universal Coordinated Time (UTC). But this is all done after the fact and only about once a month. UTC is ultimately based on the variable rotation of the Earth (hence: leap seconds), the orbit of the Earth around the Sun, and our planet's relationship to distant stars and quasars; it's determined by astronomers over a long span of time. So UTC isn't really a "real-time"... time. In the U.S., when we synchronize our clocks, it isn't really to UTC. It's probably to UTC(NIST). Or, maybe, UTC(USNO), produced by the U. S. Naval Observatory (USNO), particularly if we're in the military. Or, more realistically, GPST. Most of the clocks/NTP servers I've built are "disciplined" to GPST, adjusted to approximately UTC by adding leap seconds; this is what all the cool kids do these days.
Cesium atomic clocks are noisy in the short term (jitter) - so they are imprecise - but averaged over long durations they are extremely stable - so they are accurate. Hydrogen maser atomic clocks are extremely precise (very low short term noise) but are inaccurate - one scientist said using a hydrogen maser was a "black art" because you would never be exactly sure what extremely stable frequency you would actually get until you turned it on (weird). So NIST, USNO, and others use an ensemble of multiple commercial cesium (Cs) clocks, the long term average of which is used to servo the output of an oscillator that is locked to a hydrogen maser clock. Below is a photograph of an broken commercial hydrogen maser clock sitting in a hallway; new, they go for around a quarter million dollars U.S.
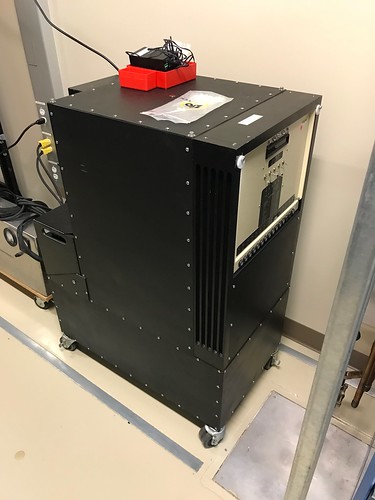
The only way to measure the quality of a clock is with another clock. This is just as problematic as it sounds, particularly since really good clocks are measurably affected by not just environmental noise like vibration, thermal noise, and even quantum noise, but also by special and general relativistic effects. We spent almost an entire day on measuring and characterizing phase noise in clocks, which is really all about measuring phase differences between a clock you trust, and one you don't, and then processing the raw data by computing an Allan Deviation (ADEV, or AVAR for the Allan Variance). Because the measurement of phase differences is a time series and not a population, the normal statistical methods don't apply. Below is a photograph of the equipment we used for a hands-on demonstration of measuring and characterizing phase differences. One of the carts contained a high-precision oven-controlled quartz oscillator that is kept running on an uninterruptible power supply so that it stays in its "sweet spot". One of them also contained an atomic clock in the form of a rubidium (Rb) oscillator.
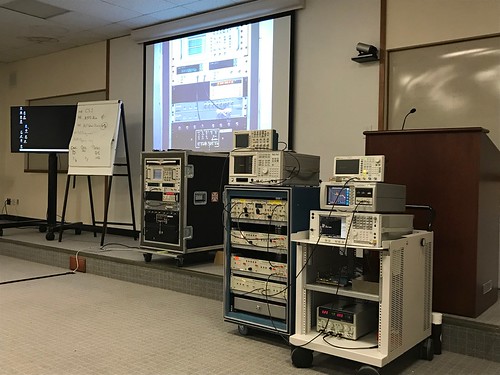
The latest "optical" atomic clocks - so called because their resonant frequency is in the visible light range instead of the microwave range like cesium or rubidium atomic clocks - have frequencies so high they can't be measured directly because electronics can't run that fast. So they have to have "optical frequency combs" that produce a lower, measurable, frequency - 10MHz or even a low as 1Hz a.k.a. 1PPS - that is locked to the higher optical frequency. Optical atomic clocks, still in the experimental stage today, are likely to eventually replace cesium atomic clocks as the definition of the second in the International System of Units (SI). That's one reason why labs at NIST may contain awesome.

One of the optical clock labs was officially surveyed to determine its position within a millimeter or so. Because an optical atomic clock is so accurate, general relativistic effects are measurable if it’s moved just a few centimeters vertically from the Earths mass. The clock depends on measurements of less than the width of a hydrogen atom. There's an official Geodetic Survey marker embedded in the concrete lab floor.
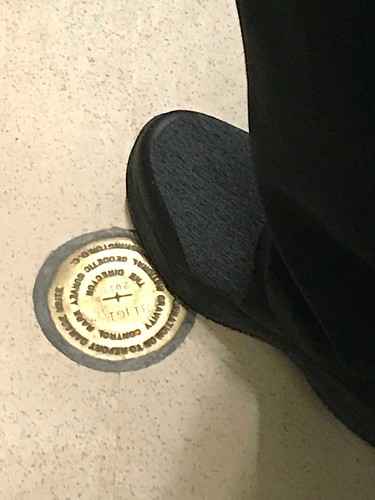
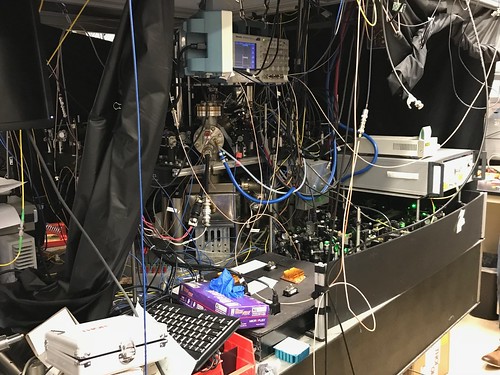
(I observe that this idea of entangling two atoms, "exciting" one and "interrogating" the other, is pretty much the same reason that historically the most accurate mechanical pendulum clocks had two pendulums, one slaved to the other.)
The latest optical clocks are so accurate and precise that they are being used to probe the "constant-ness" of certain values in physics - like the dimensionless fine-structure constant - that we think are constant, but have no theory that says why they must be constant. As we continue to make things smaller and smaller, and faster and faster, the measurement of such values will matter more and more. One of the NIST experiments involves comparing two different implementations of optical clock-type technologies and making very fine measurements of any differences in their results that may be affected by variation in electric field strengths. In the photographs below, you can see the green versus the blue LASERs, denoting different atomic resonant frequencies at use.
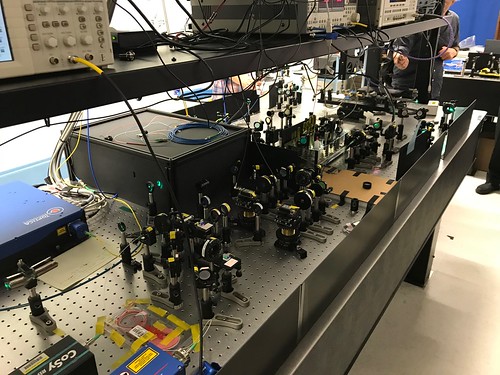
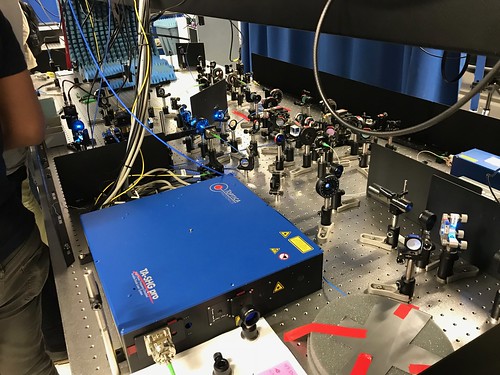
Because we can measure time far more accurately - several orders of magnitude better - than any other physical unit of measure, we can expect other SI units to eventually be defined in terms of time whenever possible. So these technologies have been used by NIST to develop measurement standards for other physical units. Below is a photograph of a ten volt (10V) reference standard made up of an array of superconducting Josephson junctions on semiconductor wafer (left) placed in carrier (right) that goes in cryogenic chamber. It's accurate to maybe twelve significant digits. One wafer is worth about US$50,000.
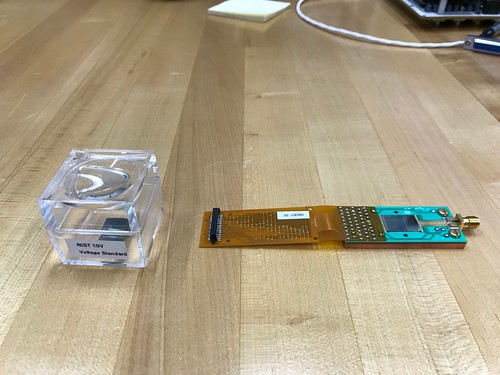
This is used in the instrument used below to calibrate volt meters. Well, maybe not your voltmeter. NIST has built quite a few of these instruments and sold them to various labs here and abroad. You can vaguely see the small cryogenically cooled chamber behind the screen in the lower left of the rack. The noise of the compressor for the cooler was a constant thumping in the room.
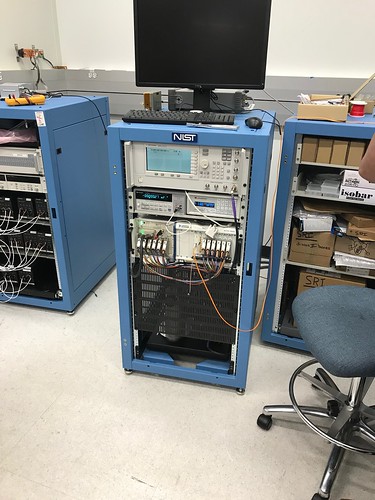
I wore a mechanical wristwatch each of the four days of this conference. It just seemed appropriate. I wasn't the only one. Another guy and I compared oscillator frequencies. Mine was twice as fast as his. I have watches with 4, 6, and 8 Hz oscillators, and even a vintage "fast beat" watch with a 10 Hz oscillator. The one I was wearing that day, shown below, has an 8 Hz mechanical oscillator. Compare that to your everyday quartz wristwatch... that typically has a 32,768 Hz quartz oscillator. Conventional atomic clocks oscillate in the gigahertz (microwave) range, while optical atomic clocks oscillate in the terahertz (visible) range. Future clocks will be "nuclear clocks", oscillating in the petahertz to exahertz (x-ray) range, "ticking" via transitions in the nucleus of an atom. I think maybe NIST wins the oscillator frequency challenge.
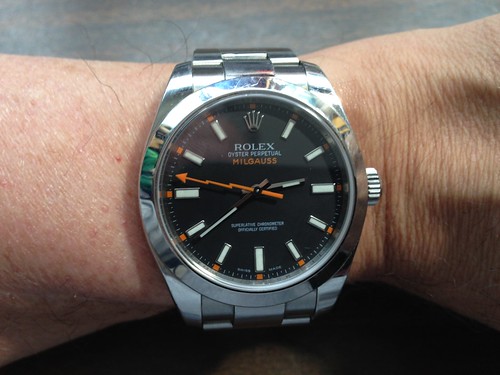
A big Thank You to NIST and its scientists that participated in the seminar and who were so generous with their time and expertise. Because, you know, ultimately, it's all about time and frequency.